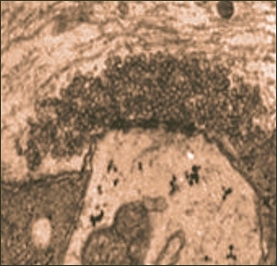 |
Synaptic vesicles the tiniest circles clump together at the membrane, ready to release neurotransmitter |
In every home, there is at least one area designated for storage. Things get haphazardly thrown in and each time something new goes in, you look at it and think, some day, I'll organize it and throw out what I don't need.
We all have similar
storage rooms in our body, except these ones we definitely
don't want to empty out. They are known as synaptic
vesicles, tiny sacs in our brains that store the chemical
information that allow messages to pass from neuron to
neuron.
Dr. James Cheetham studies how certain proteins interact with these tiny synaptic vesicles to encourage neurotransmission and stabilize these vesicles. His work offers a key to understanding how some diseases such as Parkinson's can be overcome with proteins and how drug addiction works.
His office at Carleton
University in Ottawa looks like one of those messy storage
rooms. He sits in front of two computer monitors and a
laptop; bookshelves are crammed; folders
form mountains on the corners of his desk. He displays
cartoon drawings of old bosses who have influenced his
work. "He kinda looks like Mr. Burns (from The
Simpson's)," he jokes about one of them.
Cheetham uses his
laptop's PowerPoint presentation with over 50 slides to
talk about the work he is doing. He starts by explaining
neurotransmission and how synaptic vesicles are involved
in this process.
The invisible world
Working with synaptic
vesicles involves working with incredibly tiny parts of
the brain. They are 40 nanometres in diameter. This means
it would take one million synaptic vesicles to create a
one centimetre line, Cheetham says.
'They are 40 nanometres in diameter. This means it would take one million synaptic vesicles to create a one centimetre line.' |
They are key in the
process of neurotransmission. They hold the
chemical information the neuron uses to create an
electrical signal to send to the next neuron.
Picture
neurotransmission as a set of dominoes that are placed on
their edge in a random pattern, but close enough to one
another so if one fell, the others follow. The synaptic vesicles are
the white dots on the domino.
When the first electrical
signal, (your finger), stimulates the first neuron, (the
domino), the synaptic vesicles fuse to the membrane of that
neuron and release their neurotransmitter. Then that
neuron becomes excited and sends another electrical signal
to another neuron. In essence, it's like each domino
falling over, forcing another one to do the same until the
desired action is carried out.
The electrical signal
that stimulates the first neuron is called the 'action
potential' and it moves down the axon between two neurons.
It is like a signal travelling down a telegraph line.
"The action potential either happens or does not
happen, like flushing a toilet or ringing a
doorbell," Cheetham says. "When the action
potential gets to the nerve terminal, it can cause the
release of variable amounts of neurotransmitter."
There are four main
neurotransmitters. Glutamate gives you the excited
feeling, serotonin deals with reward pathways - the
"high" feeling - GABBA is inhibitory and
dopamine deals with movement.
After the desired
neurotransmitter is released, you have to get rid of the
empty vesicles and this is done through a re-uptake
process. This is when the recycling factory kicks in and
the pre-synaptic cell, or the cell from which the synaptic
vesicles were released, sucks the used ones back up like a
vacuum cleaner so they can refuel them with neurotransmitter.
Building armies
If the body is working
hard and quickly to send messages across the brain, there
is the danger this re-uptake process can't keep pace. What
that means is the vesicles that have been emptied can't
get refuelled fast enough before they are needed again,
Cheetham says.
This is where Cheetham's
work with proteins comes into play. Synaptic vesicles form
clusters around the membrane. To carry out a normal
action, the front-line vesicles would be released, then
sucked back up, refuelled and sent back out. But if a
long-term action is required and the re-uptake process
can't keep up with the demand, the cluster acts as a back
up army.
Instead of firing, then refuelling and firing the same vesicles again, this back
up army supplies the front lines with
neurotransmitter-full synaptic vesicles. But without
proteins, this back up army wouldn't exist.
Because synaptic vesicles
are so tiny, their membranes are stretched to the limit.
Cheetham says when membranes curl that tightly, they have
a tendency to want to join together to make a bigger
membrane. Proteins help stop this from happening.
Cheetham has also found,
through his work with a certain family of proteins called
synapsins, that the proteins help keep the vesicles
clustered together so the backup army is there. Without
the backup army, the brain doesn't get enough
neurotransmitter to carry out a longer-term desired
action, he says.
Mapping proteins
"If you know how a
protein works on synaptic vesicles, it gives you a better
idea of how synapses work. This gives you a better
understanding of the molecular basis for diseases. If you
know that, you can develop ways to counteract it,"
Cheetham says.
Ryan Doherty is a fourth
year undergraduate student at Carleton. He works in
Cheetham's lab developing three-dimensional models of
proteins from the synapsin family by mapping out the protein's sequence.
This gives them both
insight into how that protein may be of
use and how it interacts with other proteins and
neurotransmitters. For example, you can map out the region
of the protein that could bind to the lipid bilayer of a
cell, Doherty says.
The lipid bilayer is a
membrane with two levels of lipid cells (commonly referred
to as fat cells) which combine with proteins and
carbohydrates to make up the structure of the cell. It's
like drawing a circle in a circle - the double outline is
your lipid bilayer.
With this information,
Cheetham has been able to discover the way in which
synapsins target and act on synaptic vesicles and the
interaction these proteins have with the lipids in the
cell. Because he knows how the protein family of synapsins
interacts with the lipid membrane of a cell, he can tell
how the protein will stabilize the vesicles inside the
cell.
Doherty says "once
you understand the interactions, you can make a model that
will help in looking at, for example, how drugs interact
with proteins to cure diseases." Then you can model
drugs to target the specific parts of the protein that
will have the desired effect.
Targeting diseases
This kind of work is being used in researching and understanding Parkinson's disease. "The neurons that produce and use dopamine as a neurotransmitter (dopaminergic neurons) in the basal ganglia (a part of the brain involved in controlling movement) selectively die off," Cheetham says.
In some people, this happens faster than in others which means they can't produce enough dopamine to regulate their movements.
Cheetham's work could one day provide the needed information to tell us how proteins can be targeted to help the cell produce more dopamine, but he says right now, he is not very close to having these techniques used for medical purposes.
Currently, to treat Parkinson's disease, doctors are injecting stem cells, cells which have not yet designated themselves to a given role in the body, into the basal ganglia so they will become dopamine-producing cells. More commonly, they just inject dopamine into the body.
Dopamine is just one of the four main neurotransmitters previously mentioned. Another is serotonin, the neurotransmitter that stimulates the reward pathways in the brain. Synaptic vesicles are therefore instrumental in drug users' "highs".
Highs and lows
The drugs cocaine and ecstasy inhibit the re-uptake of serotonin, Cheetham says. So when it is released, it stays in the system and the user gets a prolonged 'high' feeling. This can easily lead to drug addiction.
The more you use a certain pathway, whether it be by playing the same piece of music on a piano, or taking the same drug over and over again, the pathway used to carry out the action strengthens. This is a process known as long-term potentiation.
This principle applies to drug users in that by taking a drug, the re-uptake process fails. The pathways that use serotonin are therefore strengthened by the excess chemical, so you have to take more of the drug to get the same high. In other words, the user builds up a tolerance.
Eventually, what happens is the user over-stimulates the serotonin pathways and the neurons shut down, Cheetham says. If you know how this works, you can intervene with medications, he adds.
Synaptic vesicles are therefore involved in everything we do be it turning a page in a book or running a marathon. Without them, the brain can't continue to send messages through the body to continue a desired action.
These vesicles are also involved in how certain diseases such as Parkinson's effects the body and how drug addiction works.
Exciting work
Cheetham is not alone in studying synaptic vesicles. He as been at Carleton for six years, but studies in how proteins effect synaptic vesicles have been going on for around thirty years, he says. In the November 17, 2001 issue of ScienceDaily Magazine, scientists discussed how a protein can be used to mature synapses, the gaps between neurons over which the electrical signal must pass to transmit information.
The protein, dubbed PSD-95 "helps build the physical scaffolding of the synapse that cells use to transmit the chemical messenger . . . glutamate." Glutamate is also an excitatory neurotransmitter. In this case, by maturing the synapse, the protein allows for an increased number of synaptic vesicles to adhere to the membrane and thus increases the number of them that release their neurotransmitter, so more neurotransmitter is released.
According to the article, "glutamate enables the brain to develop, language to be learned, a new math equation to be grasped, tennis to be mastered and walking to be relearned following a stroke. But without the synapses that allow the chemical signal's transmission from one nerve cell to the next, glutamate has no more luck in communicating its messages than a train has luck in reaching its destination without tracks to follow."
This research was important in learning about how to strengthen the pathways and their ability to transmit a stronger signal in order to help treat mental retardation which is caused when the post-synaptic cell can't receive the glutamate messages effectively.
Research continues in the area of synaptic vesicles and how proteins interact with the vesicles to create different outcomes. Not only have scientists found ways to mature the synapses to allow for stronger neurotransmission, but Cheetham has found how the protein family synapsins stabilize synaptic vesicles and prevent them from binding together when they shouldn't.
With the PowerPoint screen finally blank, Cheetham leans back in his chair and contemplates the question of "where to next?"
"You just keep on going," he says.
Scientists working in this area have a vast array of proteins to chose from. Cheetham has just been working with one family.
"There is so much, you never run out of things to work on," he says.
|