Mimicry in action
By Michelle Catton
OTTAWA —
Mimics come in many forms.
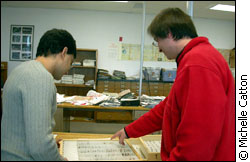 |
Tom Sherratt and his research
student Arash Rashed study hoverflies to learn about
natural mimicry. |
Male fish pretend to be females to improve
their chances of reproduction, non-poisonous beetles
pretend to be poisonous by raising their backsides in
the air and making idle threats to predators, and flies
pretend they are wasps to avoid being eaten.
Batesian mimics, named after British naturalist
Henry Walter Bates who first observed them in the mid-nineteenth
century, are nature’s cheaters, piggybacks on
the success of other species.
Their story begins innocently enough.
It starts with two species, such as one type of butterfly
and one type of moth, each hunted by the same predator.
The butterfly, like the Monarch, eats
milkweed plants while the moth feeds on different plants.
Since milkweed is poisonous and distasteful to birds,
it makes the monarch unpalatable for its avian predators.
“Many organisms in nature are defended
in some way. They are poisonous, or nasty tasting, or
they have a bite or sting to deter predators. It’s
better for these organisms if they can advertise themselves
somehow, to say to the predators – hey, don’t
eat me,” explains Christopher Beatty, a PhD student
in behavioural ecology.
The Monarch butterfly next develops a
brightly coloured pattern to warn predators of its poisonous
nature, the beautiful orange and black design we see
today. This is called the development of a warning signal,
nature’s stop sign, telling predators to keep
away.
The moth is now at a disadvantage. Not
only is the moth a tasty treat for birds, its competitor
for survival is advertising to predators its unpalatability,
making the moth particularly vulnerable.
The birds have learned to avoid brightly
coloured butterflies, so through natural selection,
moths with bright colours resembling the Monarch live
longer and produce more offspring than those with no
pattern or colour.
Eventually, the moth develops a black
and orange design strikingly similar to the Monarch
butterfly. Even though it isn’t defended in any
way, it uses the Monarch’s pattern as a shield,
tricking predators into thinking it’s distasteful.
'People might
not think you could go fishing for dragonflies' |
Arash Rashed, one of Sherratt’s
PhD students, sits at the computer closest to the window.
He is waiting, perhaps more eagerly than the rest of
us, for spring to arrive in Ottawa. With the onset of
warm weather, he can continue the field research he
started last year, the study of the evolution of Batesian
mimics, or copycats.
“I always have to wait for summer
to do my experiments,” laments Rashed, who is
examining the effects of insects on the evolution of
mimicry, specifically dragonflies.
Although his research interests sound
impressive, his experiments are less than glamourous.
For days on end, Rashed stands in a field holding a
stick above his head, dangling flies and bees in an
attempt to entice predators.
“People might not think you could
go fishing for dragonflies,” laughs Rashed, who
developed strong arm muscles after “fishing”
for six hours a day all summer long.
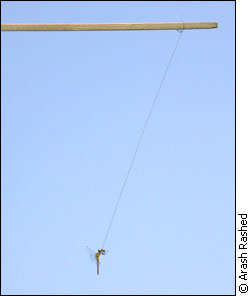 |
Rashed fished for dragonflies
using hoverflies and bees as bait last summer. |
Rashed is studying an area of evolutionary
theory that has rarely been examined. Scientists have
identified birds as the predators that influence the
evolution of mimicry, but Sherratt and Rashed think
insect predators, like dragonflies, can also play a
role. They are examining whether or not they avoid eating
brightly coloured, patterned species.
“Invertebrate (insect) predators
can play a role in evolution as well. We wanted to know
what role dragonflies play,” says Rashed.
They started with bees and hoverflies.
Hoverflies are closely related to black flies, but they
are bee mimics. They have the same body shape and black
and yellow pattern as bees or wasps. Because hoverflies
are such good mimics, humans can barely tell the difference
between the two. Rashed wanted to know if dragonflies
could sense something humans can’t.
First, he captured bees and hoverflies
with insect nets, then hung one of each on strings descending
from his stick. He recorded the results of his “fishing”
— how often a dragonfly ate the bee offering compared
to the number of times it chose the hoverfly.
“We saw a small tendency for the
dragonflies to go for the mimics (hoverflies), but since
it was very slight, we can’t be sure,” says
Rashed. “Other studies have found that dragonflies
avoid black and yellow in different species altogether.”
Because their findings are contrary to
those published in the past, Sherratt and Rashed have
decided to repeat their experiment this summer. They
want to make sure their results are correct before they
release them to the scientific world.
Although Rashed is eagerly awaiting spring
and the chance to resume his field work, he keeps considerably
busy over the winter months. In fact, he and Sherratt
have pioneered a computer program that may change the
way mimics are investigated forever. They call their
program Neural Networks.
'Sometimes, mimicry
is not perfect, and we want to take that into account' |
“Now, we have no way to objectively
measure the similarity between mimics and their models.
Sometimes, mimicry is not perfect, and we want to take
that into account,” says Rashed.
A problem with mimicry field work done
around the world is evaluating mimics. When Rashed chose
hoverflies for his experiment, they all seemed like
good mimics to him, but in fact, some hoverflies resemble
bees more than others. Although the human eye may not
be able to tell the difference, dragonflies probably
can. Up until now, there was no way for humans to differentiate
between a poor mimic and a near-perfect mimic. The Neural
Networks computer program could change that.
“The system measures the antenna
length, colour, length of wings, etc. of photographs
of mimics and their models. We train the system to tell
us how closely the mimic resembles the model,”
says Rashed, whose program spits out a number to tell
the researcher how good the mimic actually is. A near-perfect
hoverfly can be close to 99 per cent similar to a bee,
while a poor mimic might only be 50 per cent similar.
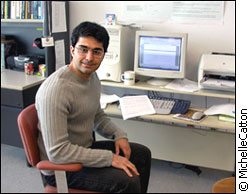 |
Rashed works on a mimicry
computer program during the winter months. |
Although the program is still in its beginning
stages, Rashed and Sherratt are bolstered by comparisons
with animal experiments. A study done at the University
of Nottingham, in the United Kingdom, trained pigeons
to differentiate between poor mimics and near-perfect
mimics.
Their method was similar to that employed
by Rashed’s computer program. They trained one
set of pigeons to identify pictures of flies by rewarding
correct identifications. They trained another set to
identify bees. Then they presented all the pigeons with
pictures of hoverflies, and recorded how often they
were correctly identified as flies by the fly-trained
pigeons, and how often they were mistakenly identified
as bees by the bee-trained pigeons. They recorded percentages
similar to those produced by Rashed’s program.
“The pigeons identified what looked
like a poor mimic to humans as a near-perfect mimic.
The computer program did the same thing,” says
Rashed. This suggests his program can think like a pigeon,
a predator, when humans can’t. The technology
could be used by scientists all over the world investigating
the evolutionary theory behind mimicry.
'It happens in
the Amazon with poisonous frogs. Different frog
species with the same predator are all the same
colour. It makes sense for survival' |
Christopher Beatty, who occupies a computer
in the dusty, windowless corner of Sherratt’s
lab, is working at the other end of the mimicry spectrum.
While Rashed is examining detailed ways of evaluating
mimics, Beatty wants to understand the basic systems
behind the phenomenon. He uses humans as predators,
monitoring their behaviour to simulate natural conditions.
He looks for generalizations about mimicry.
“We’re not trying to evaluate
human psychology,” says Beatty.
The phenomenon Beatty is evaluating is
called Müllerian mimicry, named after a German
zoologist. It happens when two or more species, both
poisonous or defended, develop the same brightly-coloured
pattern to warn predators away.
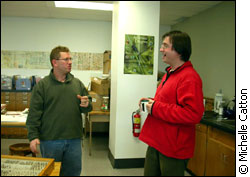 |
Beatty and Sherratt discuss
Mullerian mimicry in their lab over morning coffee. |
"It happens in the Amazon with poisonous
frogs. Different frog species with the same predator
are all the same colour. It makes sense for survival,”
says Beatty.
For warning signals to work, predators
must learn that a particular colour or pattern should
be avoided. They have to eat one or two frogs before
they learn. If all the frogs had different patterns,
a predator would have to eat several of each variety
before they learned them all. By maintaining a similar
warning signal across species, like bright blue skin,
a predator only has to eat one blue-skinned frog of
one species before it learns that all blue frogs are
bad.
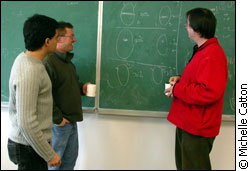 |
Rashed, Beatty, and Sherratt
examine data to uncover the mystery of mimicry evolution. |
While Rashed fishes for dragonflies to
test his theories, Beatty fishes for students to test
Müllerian theory. He hovers in the library with
his laptop, asking students to play his evolutionary
computer game. The students hunt for computer-generated
prey using a mouse, getting points for “eating”
non-poisonous victims, and losing points when eating
poisonous ones. Prey vary in their conspicuousness,
and their physical traits are passed on to their digital
offspring. In this way, Beatty can evaluate how warning
signals evolve over time, and how they are passed on
from generation to generation.
“We want to know how they get over
the hump genetically, how they all develop the same
warning signal,” says Beatty.
Most of the theories Beatty tests have
been long-accepted in the scientific community, but
because evolution is naturally slow, it is hard to observe
it in nature. Beatty’s program allows these theories
to be tested quickly and with relative accuracy.
'We think –
isn’t this the most logical way for this to
work? But we have proven that this is the way it
definitely works' |
“We know it’s artificial to
use humans as predators, but we are examining relatively
simple systems,” qualifies Beatty. “To a
certain extent, it makes sense.”
After examining their data, Sherratt and
Beatty have found that defended prey, those with warning
signals, move slower and more erratically than undefended
prey, giving predators more time to recognize their
stop signs. This explains the dizzy flight pattern of
the Monarch butterfly. They also found that defended
prey evolve warning signals for the sole purpose of
making undefended prey vulnerable.
“We find our results funny,”
says Beatty. “We think – isn’t this
the most logical way for this to work? But we have proven
that this is the way it definitely works.”
Sherratt has a reputation as a theorist.
His contribution to mimicry theory over the past decade
has been considerable.
“He's an unusual combination of
an excellent fieldworker and a researcher with significant
mathematical and modelling skills - just the job in
today's cut-throat academia,” says Francis Gilbert,
a researcher in the U.K. also studying hoverflies. “In
my view, his work has made most of the really important
recent advances in the field, a field that has been
more or less stagnant for decades.”
Although his work has few short-term practical
applications, Sherratt says it’s important to
know as much as possible about the natural world.
“It’s helpful to understand
what makes evolution tick,” says Sherratt.
|